La tDCS monopolare potrebbe influenzare i riflessi del tronco encefalico: Uno studio computazionale e neurofisiologico
Monopolar tDCS might affect brainstem reflexes: A computational and neurophysiological study
Autori
Guidetti Matteo [‘‘Aldo Ravelli” Center for Neurotechnology and Experimental Brain Therapeutics, Department of Health Sciences, University of Milan, Milan, Italy]
Anna Maria Bianchi [Department of Electronics, Information and Bioengineering, Politecnico di Milano, Milan, Italy]
Marta Parazzini [Institute of Electronics, Computer and Telecommunication Engineering, CNR, Milan, Italy]
Natale Maiorana [‘‘Aldo Ravelli” Center for Neurotechnology and Experimental Brain Therapeutics, Department of Health Sciences, University of Milan, Milan, Italy]
Marta Bonato [Institute of Electronics, Computer and Telecommunication Engineering, CNR, Milan, Italy]
Rosanna Ferrara [‘‘Aldo Ravelli” Center for Neurotechnology and Experimental Brain Therapeutics, Department of Health Sciences, University of Milan, Milan, Italy]
Giorgia Libelli [‘‘Aldo Ravelli” Center for Neurotechnology and Experimental Brain Therapeutics, Department of Health Sciences, University of Milan, Milan, Italy]
Alberto Priori [‘‘Aldo Ravelli” Center for Neurotechnology and Experimental Brain Therapeutics, Department of Health Sciences, University of Milan, Milan, Italy]
Tommaso Bocci [‘‘Aldo Ravelli” Center for Neurotechnology and Experimental Brain Therapeutics, Department of Health Sciences, University of Milan, Milan, Italy]
Introduction
Transcranial direct current stimulation (tDCS) has recently gained great interest due to its feasibility and available clinical evidences1. Several findings 2,3 suggest it can generate significant electric fields (EF) in subcortical regions, possibly modifying their activity. Several authors have tried to steer the EF to target deeper brain structures by setting number and position of scalp electrodes – a strategy called multi-electrode tDCS4,5. For example, monopolar montages (i.e., with reference over right deltoid) might induce greater concentration of currents6 and greater intensities of EF in thalamus and midbrain, compared to cephalic montages7. A way to evaluate the effect of the stimulation on brainstem is to assess trigeminal reflexes (e.g., blink reflex – BR, masseter inhibitory reflex – MIR), whose arcs relies on brainstem neural circuits (pons, medulla oblongata)8,9. In this study, we aimed to assess whether a monopolar multi-electrode tDCS (anodes over motor cortices, cathode over right deltoid) montage might selectively affect deep brain structures through computational predictions and neurophysiological assessment of BR and MIR.
Methods
A MRI-based human head model10 was used to model the EF induced by the experimental protocol of stimulation. Sponge electrodes were modelled, with a uniform electrical potential (± 1 V) over the surface. The peak (99th percentile), median, 25th and 75th percentile of the EF amplitude distribution in thalamus (THA) and mid-brain (MB), and the percentage of area where EF amplitude was greater than 25% (V25), 30% (V30), and 50% (V50) of the peak in the grey matter were considered. All values were normalized to the 99th percentile of EF in the grey matter. Then, the experimental protocol of stimulation (single session, 2 mA for 20 min, anode rubber pads: 25 cm2, reference pad: 35 cm2) was applied to 10 healthy subjects (mean ± SD age: 31.5 ± 9.7, 5 women). BR (reflex threshold, latencies of RI, RII ipsilateral and RII contralateral) and MIR (onset latencies and duration of SP1 and SP2) characteristics were recorded before (T0) and after (T1) the stimulation. Computational estimations were reported descriptively, while neurophysiological data were analysed through paired t-Test (T0 vs T1) to assess the effect of the treatment (p-value < 0.05 set as significant).
Results
Computational predictions suggest that the experimental protocol of stimulation might induce normalized medians (MB = 0.34; THA = 0.39), 25th (MB = 0.27; THA = 0.32) and 75th (MB, THA = 0.45) percentiles in deeper regions roughly comparable to those in the grey matter, with peak values always above ~70% of the peak in the grey matter (fig.1 and fig.2). Similarly, values of volume percentage suggest a concentration of EF for MB (V30 = 62.25%; V50 = 17.62%) and especially for THA (V30 = 81.02%; V50 = 17.18%) (fig.3). As for neurophysiological assessments, a significant reduction in latency (ms) of RI [right BR: t(9) = 5.24, p < 0.001; left BR: t(9) = 3.21, p = 0.01] and increase of SP1 duration (ms) of MIR [t(9) = -2.46, p = 0.03] was found (fig.4).
Discussion and Conclusion
In this study, we assessed the trends of EF distributions in MB and THA during a monopolar multi-electrode tDCS. Our results suggest that this montage might induce a deep and focal distribution of EF, as previously proposed11; however, none of previous studies were clinically confirmed, nor considered to use an extracephalic electrode. Since neurons in deep brain regions are directly sensitive to weak DC electric fields12, an effect similar to those induced by tDCS at cortical level might be expected. Indeed, we clinically tested computational predictions in healthy subjects. Clinical findings seem to confirm that monopolar multi-electrode tDCS induces changes in BR, suggesting a neuromodulatory effect on brainstem. We can hypothesize that tDCS may interfere with diencephalic nuclei (e.g., reticular formation, locus ceruleus) strictly connected to pontine and medullary areas from which BR originate13. The translation potentiality of these results is great, and the possibility to directly influence brainstem activity would be of great value for many clinical applications, e.g., rehabilitation of cranial nerves disfunctions or Parkinson’s disease.
REFERENCES
1 Lefaucheur, J.-P. et al. Clin Neurophysiol 128, 56–92 (2017)
2 Parazzini, M. et al. Bioelectromagnetics 33, 476–487 (2012)
3 Guidetti, M. et al. Biomedicines 10, 2333 (2022)
4 Dmochowski, J. P. et al. J Neural Eng 8, 046011 (2011)
5 Guler, S. et al. Journal of neural engineering 13, (2016)
6 Noetscher, G. M. et al. IEEE Transactions on Biomedical Engineering 61, 2488–2498 (2014)
7 Parazzini, M. et al. Clinical Neurophysiology 124, 1039–1040 (2013)
8 Esteban, A. Neurophysiologie clinique = Clinical neurophysiology 29, 7–38 (1999)
9 Ongerboer De Visser, B. W. et al. Brain : a journal of neurology 101, 285–294 (1978)
10 Christ, A. et al. Physics in Medicine and Biology 55, 23 (2010)
11 Khorrampanah, M. et al. Computers in Biology and Medicine 125, 103998 (2020)
12 Reato, D. et al. The Journal of neuroscience : the official journal of the Society for Neuroscience 30, 15067–15079 (2010)
13 Fearon, C. et al. Journal of Parkinson’s Disease 11, 261–269 (2021)
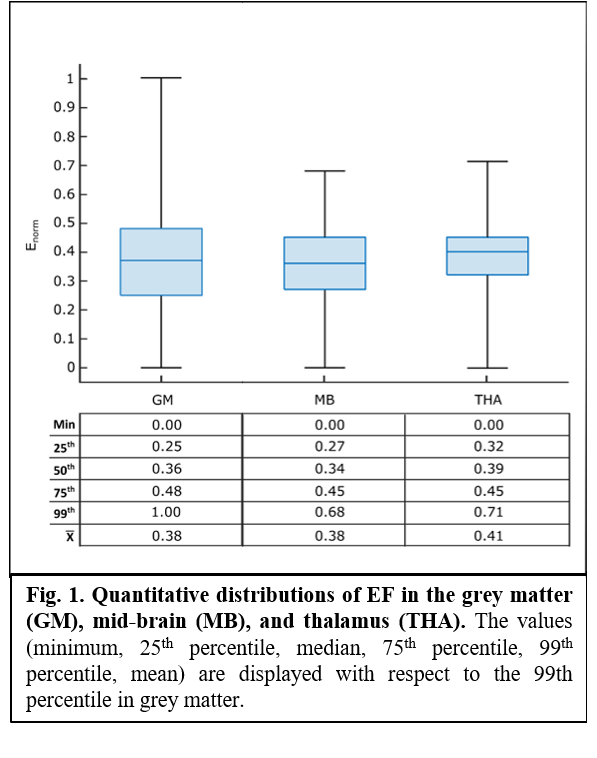
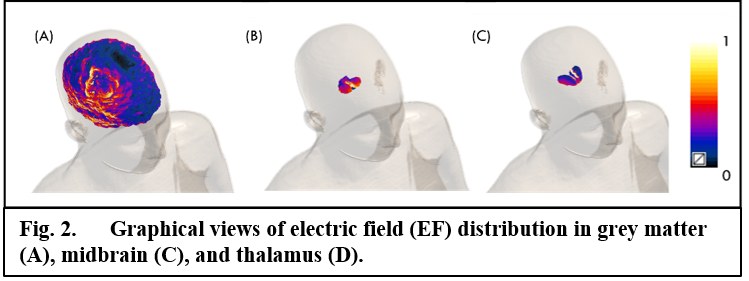
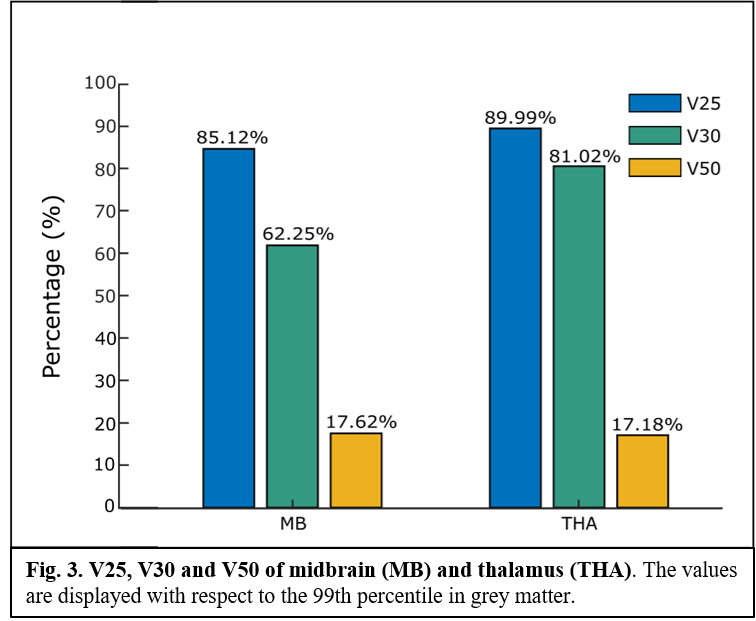
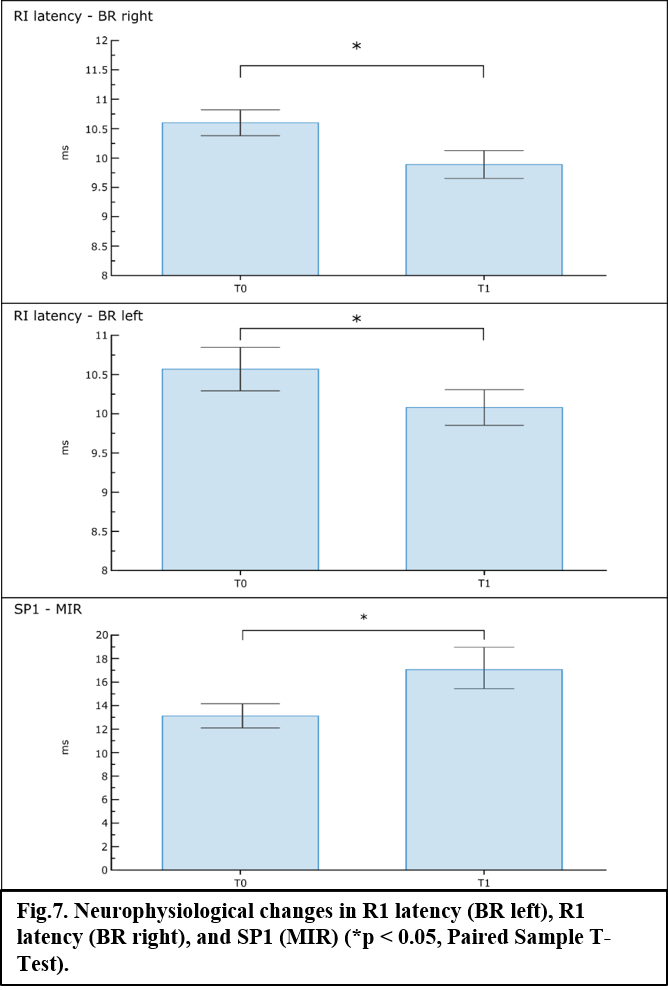